'The more we looked into the mystery of Crispr, the more interesting it seemed'
Scientists have known about the technique for years, but always assumed it was junk. Then Jennifer Doudna began to study it. Her findings could transform medicine
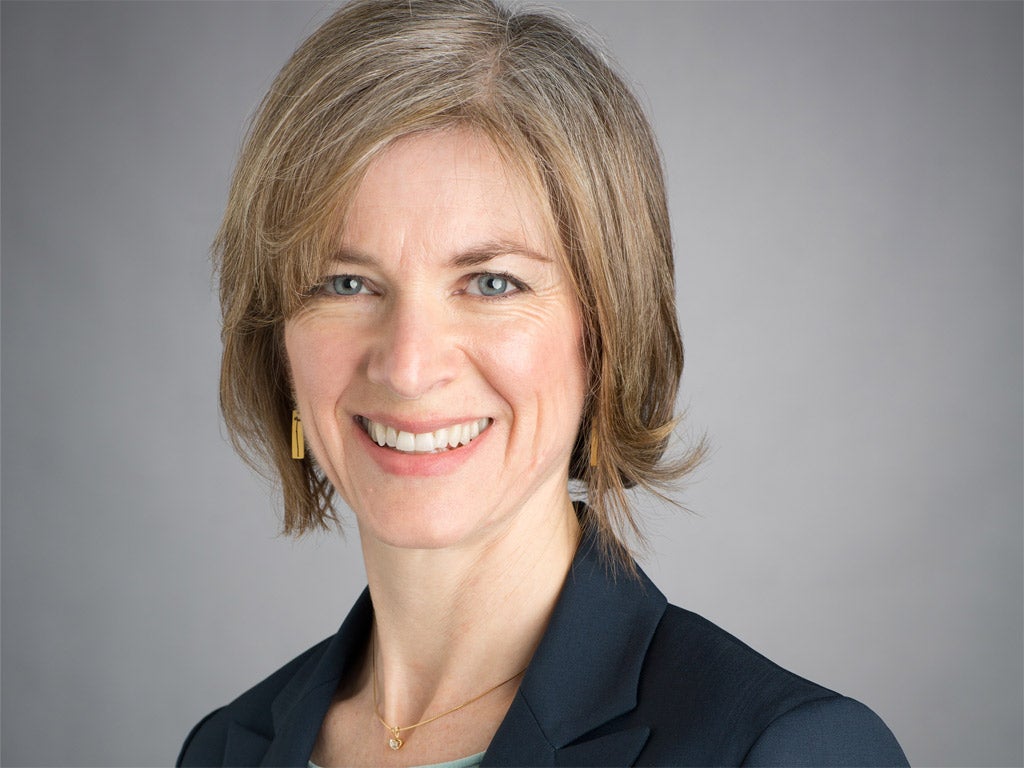
Some of the biggest discoveries in science often hide away in plain sight for many years before their importance is fully realised. This is certainly true of Crispr (pronounced “crisper”), which has taken the world of genetics by storm.
Crispr stands for “clustered regularly interspaced short palindromic repeats”, a devilishly contrived acronym which just about sums up why it was ignored for so long. For nearly two decades after Japanese researchers first discovered Crispr in bacteria in 1987, scientists mostly dismissed it as “junk DNA”.
In fact, the apparently nonsensical sequences within Crispr, which were repeated in palindromic order (the same backwards as forwards), did have a purpose and were far from junk. About six years ago, scientists discovered that these DNA sequences matched the genetic sequences of various viruses that attack bacteria, which led to the discovery of a sophisticated bacterial immune system.
Far from being junk, Crispr was actually a way of storing the genetic information of an invading virus in the form of a palindromic DNA sequence. The bacteria used this genetic memory to target the viral invader by chopping it up with powerful “Crispr-associated” (CAS) enzymes capable of “cleaving” its DNA molecule, just like a pair of molecular scissors.
The mystery of Crispr was finally resolved by Jennifer Doudna of the University of California, Berkeley, a specialist in RNA, the smaller molecular cousin of DNA. About seven years ago, she was asked by a university colleague to look into this genetic peculiarity of bacteria and quickly became fascinated.
“The more we looked into it, the more it seemed extremely interesting,” Professor Doudna said. Then, in 2011, she met Emmanuelle Charpentier of Umea University in Sweden at a scientific conference. Professor Charpentier told Professor Doudna of another kind of Crispr system that seemed to rely on a single gene, now called CAS9.
Both professors collaborated on the project and in August last year published what is now considered the seminal paper showing that CAS9 was an enzyme capable of cutting both strands of a DNA double helix at precisely the point dictated by a “programmable” RNA sequence – in other words, an RNA molecule that could be made to order. “We found that CAS9 has the ability to make a double-stranded break in DNA at sites that are programmed by a small RNA molecule. What was so important was that we could really show how the CAS9 protein worked,” Professor Doudna said.
“Not only were we able to work out how it worked, we were able to reprogramme it to recognise new DNA sequences. If it could be made to work in eukaryote systems – plants and animals – then you’d have a system where you could effectively decide where to produce a double-stranded break in that cell’s genome.”
It not only worked on plants and animals, it worked beautifully. Professors Doudna and Charpentier had found the holy grail of genetic engineering – a method of cutting and stitching DNA accurately and simply anywhere in a complex genome. Until now, this was carried out by modified viruses, which inserted their DNA at random, or by elaborately cumbersome techniques known as “zinc fingers” or “Talens”.
“You can actually introduce new genetic information at the site of cleavage. So it has become a powerful way of doing genetic engineering. It’s a fundamentally different way of recognising DNA target sites,” Professor Doudna said.
Other scientists showed that the Crispr-CAS9 system worked on a diverse range of organisms, from bacteria and yeast to nematode worms, fruit flies, plants, fish and mice. Earlier this year, George Church of Harvard University was the first to demonstrate that it also worked on human cells – a critical milestone – and the implications for human medicine became immediately obvious.
“I’m tremendously excited about the possibility of this discovery having a real impact on people’s lives. Maybe it will offer the opportunity to do therapeutics that we’ve not been able to do in the past,” Professor Doudna said.
“One can envisage taking cells from a patient with sickle-cell anaemia or an inherited blood disorder, and using the CAS9 system to fix the underlying genetic cause of the disease by putting those cells back into the patient and allowing them to make copies of themselves to support the patient’s blood.
“In the past, when we’ve tried gene therapy, we haven’t had tools that have allowed targeted-gene correction. This will be fundamentally different to what we’ve had in the past.”
Her team is already, for instance, working on possible ways of using the CAS9 system to disrupt the extra damaging chromosome responsible for Down syndrome, or the extra repetitive sequences of DNA that lead to Huntington disease, a lethal inherited disorder that strikes carriers of the defective gene in mid-life. “These are the kinds of genetic defects that one could envisage coming up with strategies to treat, which we haven’t really been able to do in the past,” Professor Doudna said. “What’s exciting is that you can see the potential, and it’s certainly going to drive a lot of research to try to explore it as a potential human therapeutic tool.”
Researchers are already talking about using it to change the gene that governs the protein responsible for allowing HIV to infect white blood cells. The idea is to mimic the natural “immunity” some people have to HIV, which prevents their progressing to Aids.
Another potential HIV therapy is to target the viral DNA that becomes integrated into the human chromosomes of people who are infected for life. If this viral genetic material can be disrupted, it might lead to a genuine HIV cure without the need to take antiviral drugs for life.
But perhaps the most intriguing and controversial application of Crispr-CAS9 will be the possibility of altering the genes of IVF embryos. Studies on mouse embryos show it is incredibly effective, and some IVF doctors may want to see if it can work on human embryos – which is illegal in Britain at present because it amounts to “germline” gene therapy.
Professor Doudna agreed the possibility is there, but she has concerns. “I think in principle it could be done, but I think personally I’d be uncomfortable with that,” she said. “Certainly, at this stage, I don’t think we understand it well enough. Would you be correcting one problem but introducing others?”
Join our commenting forum
Join thought-provoking conversations, follow other Independent readers and see their replies
Comments